
Tyler Ford
11/04/2021
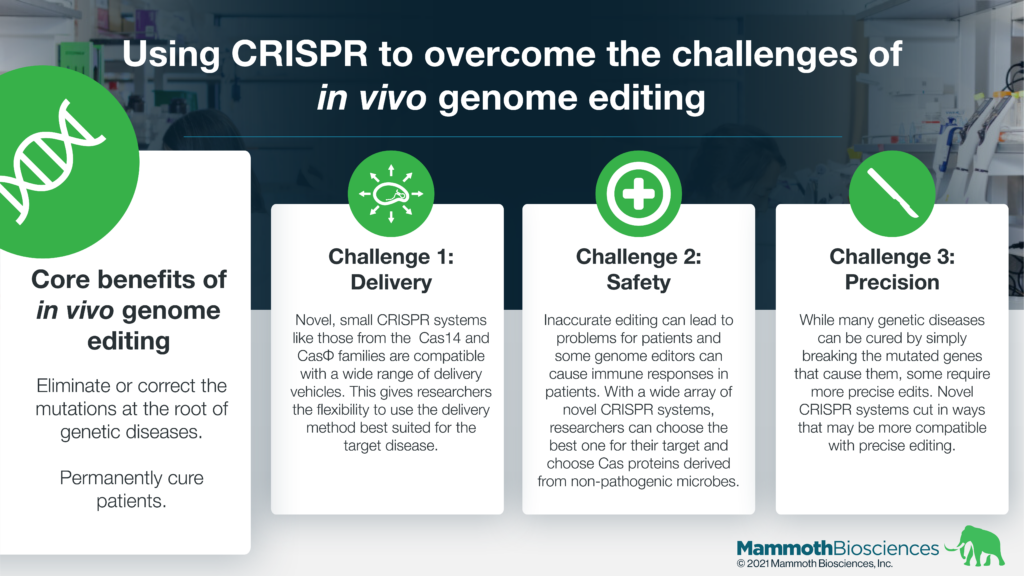
Genome editing has many applications in basic research, bioproduction, agriculture, and more. In this post, we focus on the promise of “in vivo” genome editing in healthcare.
We previously covered “ex vivo” genome editing. This involves editing cells’ DNA in the lab and delivering the cells to patients. The edited cells might replace unhealthy cells or attack diseased cells. By contrast, in vivo genome editing alters cellular DNA directly inside the body.
In the best case, ex vivo genome edited cells fight disease continuously and provide a functional cure. Yet, these cells can cause issues for patients. For example, cells designed to kill cancerous immune cells also kill healthy immune cells. Patients treated with these cells may be immunocompromised for life. Additionally, ex vivo genome editing is a lengthy process involving the use of highly specialized equipment. It often takes many weeks to produce a viable cellular therapeutic agent. This makes it unsuitable for rapidly progressing diseases.
In vivo genome editing can target the root cause of genetic diseases. It can eliminate or correct DNA mutations behind certain diseases. It can turn diseased cells into healthier or healthy cells permanently. In vivo methods may dramatically reduce the time and resources needed for treatment. As a result, in vivo methods, may make genome editing far more universal and able to target many diseases with an underlying genetic cause.
Novel CRISPR systems, like those under development at Mammoth, have the potential to expand the possibilities of in vivo genome editing. They may give researchers flexibility in their delivery methods and make it easier to target new diseases.
CRISPR-based solutions to the challenges of in vivo genome editing
The curative potential of in vivo genome editing is immense. Nonetheless, its clinical development faces a variety of challenges. Novel CRISPR systems may help researchers surmount these challenges.
- Delivery – There are many genome editing technologies. These include zinc fingers and TALENs as well as CRISPR. It can be difficult to get these genome editors into the cells they’re supposed to edit.
Researchers have been developing delivery techniques for many years. Two popular delivery vehicles are viruses and lipid nanoparticles (LNPs). Researchers have identified many factors controlling their entry into specific cells. These include the size of the cargo, clearance by the immune system, and the ability to target specific organs. Recent reports demonstrate the in vivo use of adeno-associated viruses (AAV) to deliver genome editors to the retina and the use of LNPs to deliver genome editors to the liver.
Many traditional genome editors such as TALENs as well as SpCas9 and SaCas9 are relatively large. They are difficult to deliver while Cas proteins from novel CRISPR systems like CasPhi and Cas14 are substantially smaller and have the potential to be easier to deliver. These small Cas proteins are below the payload restrictions of AAVs and enable denser packaging in LNPs. Thus, researchers may be able to deliver CasPhi and Cas14 with additional payloads. These may increase editing efficiency and make the ultimate therapeutics easier to manufacture. - Editing capabilities – It’s possible to cure some genetic diseases by breaking the mutated gene behind the disease. This is called making a “knock-out.” This is relatively straight-forward with most genome editing technologies. Other diseases need more precise edits. Novel CRISPR systems have the potential to provide advantages in generating these precise edits.
For example, some novel CRISPR systems can cut DNA in different ways than traditional systems. These different kinds of cuts may be more compatible with the precise edits necessary to treat certain diseases. Developing such CRISPR systems will make it possible to cure a broader range of genetic diseases. - Safety – Occasionally genome editors make unintended changes to target and/or non-target genetic sequences. These unintended changes can sometimes cause cells to become more diseased or diseased in a different way. For example, unintended changes might make cells better at growing and dividing. They could become cancerous. In addition, some genome editors may cause immune responses that limit their effectiveness.
Novel CRISPR systems may make in vivo genome editing safer. With access to a variety of Cas proteins, researchers may be able to choose the protein that is most likely to make the right edit without unintended changes to the genome. In addition, they can work with Cas proteins derived from non-pathogenic organisms. These may be less likely to cause immune responses in patients.
Early preclinical and clinical applications of in vivo genome editing
In vivo genome editing is making in-roads in preclinical and clinical research. In particular, researchers have had early successes with diseases of the eye and the liver. These organs are readily targeted with AAVs and LNPs.
To learn more about all kinds of CRISPR clinical trials, check out this resource from the Innovative Genomics Institute.
A future rife with genetic cures?
As researchers get better at delivering genome editors, more therapies will likely enter the clinic. The continued expansion of the CRISPR toolkit may make it easier to target more diseases and can make genetic cures more likely. These developments may ease, prevent, and end suffering for many people. At Mammoth, we’re incredibly excited to be a part of this genome editing revolution!