
Tyler Ford
01/20/2022
We’ve talked a lot about genome editing on the Mammoth blog, but there are actually many different forms genome editing can take. That is, we can make different kinds of changes to DNA depending upon what kinds of CRISPR systems and techniques we use. Indeed, different types of genome editing are more or less appropriate for different applications and for treating or curing different genetic diseases.
In this post we’ll cover 3 broad types of genome editing:
1. Knockout
2. Write
3. Modulate
Although we won’t get into the nitty gritty molecular details of each type, we will broadly define them and indicate scenarios where each could be useful.
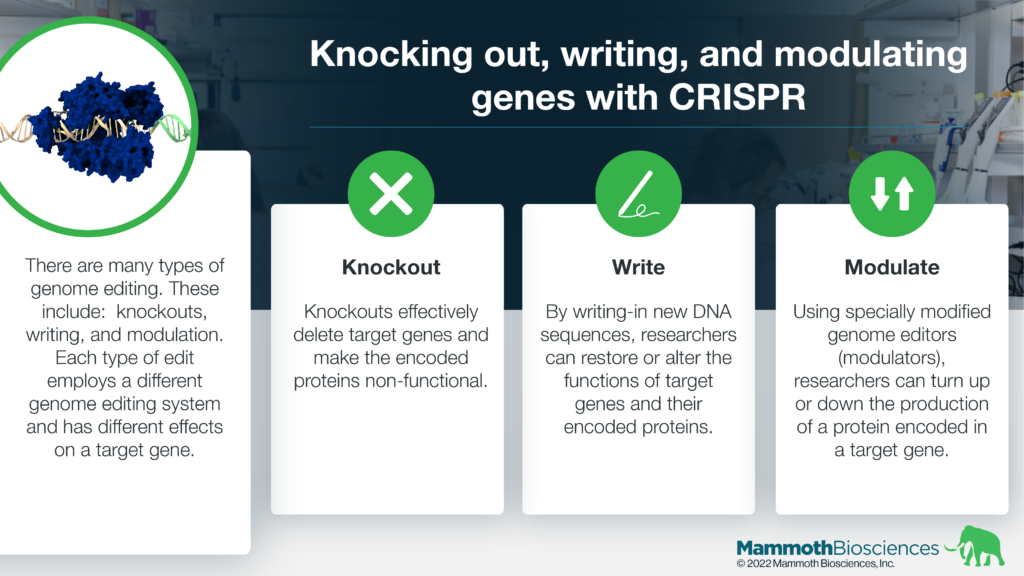
Knockout
You can think of a knockout as breaking a gene, a stretch of DNA that encodes a protein. Proteins carry out most of the functions of cells. When we knockout the genes that encode proteins, their functions are no longer performed.
Researchers can generate knockouts in a few ways:
1. They can delete the gene entirely (cut out the underlying DNA and fuse the 2 ends together).
2. They can generate a mutation that inactivates the encoded protein
(change a few of the letters in the DNA underlying the gene).
3. They can generate mutations that cause the cell to abort production of the encoded protein (usually change a few of the letters in the DNA at the start of the gene).
Often, it can be advantageous to generate a knockout by deleting the gene entirely (number 1 above). This method ensures no residual protein (whether broken or not) is created. In options 2 and 3 above, some amount of broken or truncated protein is often created. Such broken or truncated proteins can interact with other components of the cell in unexpected ways. However, it is generally more difficult to delete a gene entirely than to change a small portion of it.
Number 3 is perhaps the most practical of the options above. While this option can result in the production of residual, truncated protein, researchers can engineer their genome editors to make mutations very early in the gene. Thus only a very small truncated protein will be produced.
Knockouts can be very useful for studying protein function. To do so, researchers compare cells with the broken gene to those with the normal gene. However, knockouts are not always useful in cures for genetic diseases. Often, genetic diseases are caused by genes that are already broken. In such cases, breaking the gene further through a knockout probably won’t result in a cure. Nonetheless, there are situations where the gene behind a genetic mutation has adopted a new function that causes the disease. In these and other cases, knocking out the gene can lead to a therapy or treatment.
Write
Instead of breaking genes, we can use CRISPR technologies to make more precise changes to the DNA sequences within or around them. This is DNA “writing” or “rewriting.” This type of edit may be used to:
- Restore a mutated gene to its original form
Sometimes diseases are caused by genetic mutations that break proteins with important cellular functions. It would not be beneficial to break these genes further with knockouts. Instead, researchers can use a variety of techniques to rewrite these genes and remove the mutations. They can also use “writing” techniques to imbue other proteins with compensatory functions. - Regulate how much of protein is produced from a gene
In some genetic diseases, too little or too much of a normal or dysfunctional protein is produced from a gene. Often, the DNA just preceding or just after a gene controls how much of that protein is produced. Researchers can rewrite this DNA to adjust the levels of the encoded protein and cure disease. - Insert a beneficial gene into cells
For some therapeutic applications, like cancer and autoimmunity, our own immune cells have the ability to heal us, they just need a little help. In order to empower them, researchers can give immune cells a gene that helps beat the underlying disease. An example of this is CAR-T cell therapy. Here, researchers insert a gene encoding a chimeric antigen receptor (CAR) into a patient’s T-cells. The CAR enables the T-cells to effectively fight cancer cells.
Inserting new genes into the genome can also be exceptionally useful for studying cells in the lab. When researchers insert genes encoding fluorescent proteins like GFP or RFP into cells of interest, it is easier to visualize them. Researchers can additionally insert short marker proteins at the end of genes. This tags the encoded proteins and makes it easy to follow their life cycles in cells.
The DNA changes required for writing the genome are usually more difficult to make than those required for knockouts. However, researchers have a variety of ways of modifying CRISPR systems to make writing the genome a more efficient process. Right now, smaller changes are easier to make than larger ones, but researchers are getting better at writing the genome all the time.
Modulate
As noted above, DNA sequences surrounding a gene often determine how much of the encoded protein cells produce. While it’s possible to change these sequences to alter protein production, it’s also possible to alter protein production in an impermanent way. Although not technically “genome editing” researchers have developed a variety of means of engineering Cas proteins so they no longer alter DNA but instead increase or decrease how much the cell decodes a particular gene.
By targeting these “modulators” to specific areas of the desired gene, interactions between the engineered Cas protein and the natural cellular machinery result in the desired changes to protein production. The engineered Cas proteins do not cut DNA so their effects are not permanent. Researchers can even tweak the engineered Cas proteins so that they increase or decrease protein production to different levels.
Modulation of this kind might be desirable if a protein cannot be knocked out or re-written without causing additional detrimental effects to the cell. For example, cells might produce a protein with important functions but which also gloms onto other proteins and disrupts their functions if there’s too much of it. If cells had too much of this protein, researchers wouldn’t want to get rid of it entirely. That may cause further disease. But they could decrease the abundance of this protein with a modulator.
The impermanence of the changes caused by modulators is beneficial for a number of reasons. These include:
- If the modulator causes unintended changes to non-target genes, they won’t be permanent and will be less likely to cause issues for patients.
- The modulations cannot be inherited.
- The modulations can be made at a particular time in a cell or organism’s life when it’s most beneficial. Protein levels fluctuate throughout the life of a cell and it may only be beneficial to increase or decrease a particular protein at a specific time.
- Multiple modulations can potentially be performed simultaneously without the risks associated with cutting and editing multiple genes at the same time.
Generating the right genome edits with the right genome editor
As you can see, different types of genome edits have different uses. Indeed, because a variety of Cas proteins produce different kinds of cuts, they can and should be tailored to the kinds of alterations clinicians and researchers would like to make. At Mammoth, we’re working hard to develop an extensive Cas protein toolkit that will enable our researchers and collaborators to generate the most appropriate changes to the genome and make the most effective disease treatments and cures.