
Tyler Ford
06/08/2021
This is the second post in a two-part series on CRISPR delivery. In our previous post, we discussed some important factors to consider in CRISPR delivery. Here, we provide a broad overview of some popular CRISPR delivery methods. We break down delivery methods into physical/chemical methods and viral delivery methods. For more thorough reviews of CRISPR delivery see Wilson and Gilbert 2018, Yip 2020, Haasteren 2020, and Bulcha et al 2021.
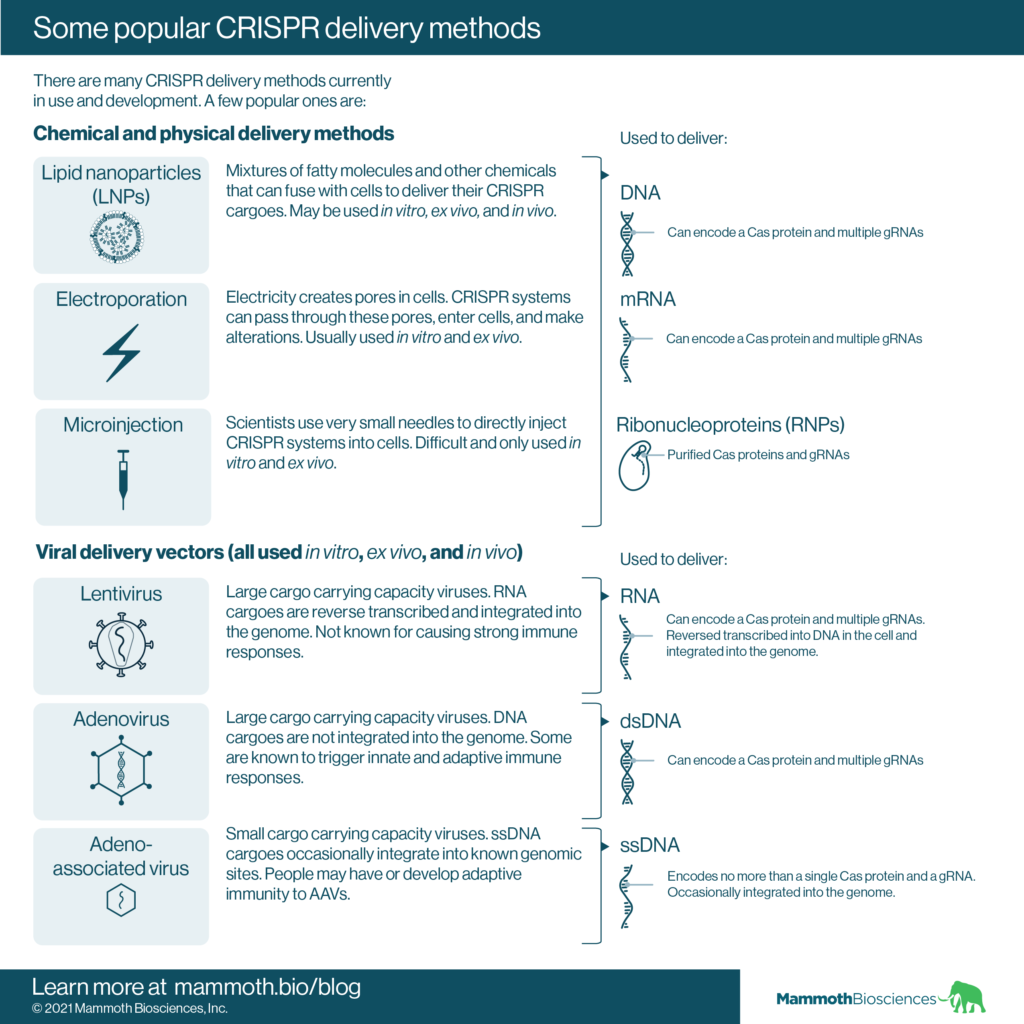
Chemical/physical delivery methods
These methods deliver CRISPR systems to cells with the aid of chemicals or devices that make cells more amenable to delivery. Some of the popular physical/chemical CRISPR delivery methods include:
- Lipid nanoparticles (LNPs) – These are mixtures of fatty molecules and other chemicals that fuse with cells to deliver their CRISPR cargoes. The specific molecules making up LNPs determine their tropism and delivery efficiency. These may be used for in vitro, ex vivo, and in vivo delivery.
Scientists have had recent high-profile successes using LNPs for the delivery of mRNA vaccines and for CRISPR delivery in mice (e.g. Qiu et al 2021, Wei et al 2020, and Rosenblum et al 2020). For an in-depth look at the development of LNPs see Kulkarni et al 2018. - Electroporation – Scientists use electricity to create pores in cells. CRISPR cargoes can pass through these pores, enter cells and make alterations to cellular DNA. Electroporation is primarily used in vitro and ex vivo.
- Microinjection – Scientists use very small needles to directly inject CRISPR cargoes into the cells of interest. Microinjection requires a lot of technical skill. Indeed, scientists perform this process under a microscope! As a result, microinjection is only performed in vitro and ex vivo. This technique is used for embryonic genome editing.
Using physical and chemical delivery methods, scientists may deliver CRISPR cargoes that take the form of:
- DNA – Scientists can create DNA encoding the essential components of their CRISPR systems. Then, they can incubate target cells with the DNA and chemicals and/or electrical pulses that make the cells more permeable. Once the DNA enters cells, the cells decode it to create things like Cas proteins and guide RNAs.
DNA is relatively easy to work with compared to RNA and RNPs (discussed below), but may integrate into the target cell genome. DNA may also direct prolonged CRISPR system expression. Both of these may lead to unwanted changes in target cells. - mRNA – Scientists can synthesize mRNA encoding their CRISPR systems and deliver this mRNA to target cells using chemicals and/or electrical pulses. The cells will then translate this mRNA to create things like Cas proteins.
One advantage of mRNA is that it is short-lived and should degrade over-time. Thus, production of the CRISPR system should cease once the mRNA degrades. In addition, mRNA does not integrate into the target cell genome. One downside of mRNA is that it is more difficult to work with and prone to degradation. - Ribonucleoproteins (RNPs) – Scientists can also use chemical/physical delivery methods to deliver purified Cas proteins and guide RNAs (RNPs) directly to their target cells.
Like RNA delivery, RNP delivery has the advantage that RNPs are short lived. In addition, RNPs cannot integrate into the genome. However, RNP delivery requires purified Cas proteins and guide RNAs. The purification process might be difficult or time consuming.
Viral delivery methods
These methods use modified viruses to deliver CRISPR system-encoding DNA or RNA directly to target cells. Viral delivery methods are used in vitro, ex vivo, and in vivo. Some popular viruses for CRISPR delivery include:
- Lentiviruses – lentiviruses can infect many different cell types and, after infection, their RNA cargoes get reverse transcribed into DNA and integrated into the genome. They can hold roughly 9,000 bases of cargo – more than enough to encode most CRISPR systems. For an in-depth look at lentiviruses as gene delivery vehicles, see Milone and O’Doherty 2018.
- Adenoviruses – Adenoviruses can also infect many different cell types, but, unlike lentiviruses, their DNA cargoes do not integrate into the genome. Adenoviruses can hold roughly 8,000 base pairs of cargo – more than enough to encode most CRISPR systems. Unfortunately, patients may have pre-existing adaptive immunity to adenoviruses. Adenoviruses elicit innate immune responses as well. For an in-depth look at Adenoviruses as gene delivery vehicles, see Gonçalves and de Vries 2003 and Lee et al 2017. Adenoviral vectors have recently been used to create COVID-19 vaccines.
- Adeno-associated viruses (AAVs) – AAVs can infect many different cell types. AAVs occasionally integrate their cargoes into specific places in the genome and have low cargo carrying capacity; they generally hold less than 4,500 bases of cargo. This is not enough capacity to carry all CRISPR systems. Patients may also have pre-existing adaptive immunity to AAVs. Finally, patients may develop resistance to AAVs after their first administration. For an in-depth look at AAVs as gene delivery vehicles, see Wang et al 2019.
Although all of these methods have their own pros and cons, scientists are actively working to improve them and devise new ones. With these techniques at their disposal, scientists are using CRISPR to learn more about a variety of biological systems and curing diseases. It’s incredibly exciting to see what the future has in store!